International Synthetic and Systems Biology Summer School
Biology meets Engineering and Computer Science
15 - 19 June 2014, Taormina - Sicily, Italy
Recent advances in DNA synthesis have increased our ability to build biological systems. Synthetic Biology aims at streamlining the design and synthesis of robust and predictable biological systems using engineering design principles. Designing biological systems requires a deep understanding of how genes and proteins are organized and interact in living cells: Systems Biology aims at elucidating the cellular organization at gene, protein and network level using computational and biochemical methods.
The Synthetic and Systems Biology Summer School (SSBSS) is a full-immersion course on cutting-edge advances in systems and synthetic biology with lectures delivered by world-renowned experts. The school provides a stimulating environment for doctoral students, early career researches and industry leaders. Participants will also have the chance to present their results, and to interact with their peers, in a friendly and constructive environment.
Sponsored by Autodesk, Leonardo Design Systems, Life Technologies, Novozymes.
List of Speakers
- Joel Bader, Johns Hopkins University, USA
- Jef Boeke, Johns Hopkins University, USA
- Jason Chin, MRC - Cambridge, UK
- Paul Freemont, Imperial College London, UK
- Farren Isaacs, Yale University, USA
- Tanja Kortemme, University of California San Francisco, USA
- Sven Panke, ETH, Switzerland
- Rahul Sarpeshkar, MIT, USA
- Velia Siciliano, MIT, USA
- Giovanni Stracquadanio, Johns Hopkins University, USA
- Ron Weiss, MIT, USA
- Liliana Wroblewska, MIT, USA
- Michael Liss, Thermo Fisher - Life Technologies, USA
- Carlos Olguin, Autodesk Inc., USA
Industrial Panel
3rd International Synthetic Yeast Genome (Sc2.0) Meeting
Taormina, Hotel Villa Diodoro Congress Center Friday, June 20, 2014 From 9:00 to 20:00Organizers and Chairs: Jef D. Boeke, Giuseppe Nicosia & Giovanni Stracquadanio
Courses
-
-
Lecture I: Elementary Circuits in Biology
To understand biological systems, our lab has defined "network motifs": basic interaction patterns that recur throughout biological networks, much more often than expected at random. The same small set of network motifs appears to serve as the building blocks of the circuitry that processes information from bacteria to mammals. Specific network motifs may be universal building blocks of biological computation. We experimentally studied the function of each network motif in the bacterium E. coli using dynamic fluorescent measurements from living cells. Each network motifs can serve as an elementary circuit with a defined function: filters, pulse generators, response accelerators, temporal-pattern generators and more. Evolution seems to have rediscovered the same motifs again and again, perhaps because they are the simplest and most robust circuits that perform these information-processing functions.
-
Lecture II: Evolution and Optimality of Gene Circuits
Organisms, tissues and molecules often need to perform multiple tasks. But usually no phenotype can be optimal at all tasks at once. This leads to a fundamental tradeoff. We study this using the concept of Pareto optimality from engineering and economics. Tradeoffs lead to an unexpected simplicity in the range of optimal phenotypes- they fall on low dimensional shapes in trait space such as lines, triangles and tetrahedrons. At the vertices of these polygons are phenotypes that specialize at a single task. We demonstrate this using data from animal and fossil morphology, bacterial gene expression and other biological systems.
-
Lecture I: Elementary Circuits in Biology
-
-
Lecture I: Network Remodeling during Development and Disease
Biological networks change in response to genetic and environmental cues. Changes are reflected in the abundances of biomolecules, the composition of protein complexes, and other descriptors of the biological state. Methods to infer the dynamic state of a cell would have great value for understanding how cells change over time to accomplish biological goals. We describe methods that predict the dynamic state of protein complexes in a cell, combining dynamic information from mRNA transcript profiling with underlying protein-protein interaction networks.
-
Lecture II : Gene and Pathway Analysis of Genome-wide Association Studies
While most genome-wide association studies are based on analysis of individual variants, the causal variants often cluster in a small set of genes. We describe Bayesian methods that exploit gene-based and pathway-based clustering to improve the search for disease-related genetic variation.
-
Lecture I: Network Remodeling during Development and Disease
-
-
Lecture I: Genome Design and Synthesis
Genome Design and Synthesis strategies vary considerable. Most of the published work on genome synthesis involves resynthesis of the native or near-native genome of bacteria. In this case, the design considerations are rather minimal and the major challenges involved have to do with design of an assembly strategy that is effective. Hierarchical strategies involve going from 10s of bases in oligonucleotides up to several megabases of dsDNA. A wide variety of methods have been described that can be used to assemble DNA and the details of these methods have significant impacts on speed of synthesis, cost of synthesis, and accuracy of synthesis. Innovative methods have been described that operate at all different levels of the assembly hierarchy. When more radical designs that deviate from that of wild-type genomes are considered, appropriate design software tools are needed. Our group has focused on genome editing software which takes advantage of well-developed browser platforms for visualizing genome features, and adapting them to genome editing. A variety of design features were somewhat arbitrarily selected for incorporation into Sc 2.0, the synthetic yeast genome. These, as well as possible future more aggressive designs under consideration will be discussed, as will interesting targets for future genome synthesis projects.
-
Lecture II: Combinatorial DNA Assembly methods and their Applications
A wide variety of pathways are available for engineering to make useful products or encode interesting behaviors in cells and organisms. As more and more complex pathways and gene networks are discovered and dissected, more and more efficient means of assembling pathways are needed. Even more importantly, libraries of these, in which the promoter strength or host of origin for the coding sequences can be systematically varied are especially valuable. Methodologies and strategies for accomplishing this are discussed, incorporating Golden Gate, MoClo, Gateway cloning, and combinations of such methods will be discussed. Examples of pathway optimization will also be reviewed.
-
Lecture I: Genome Design and Synthesis
-
-
Lecture I: Reprogramming the Genetic Code
The information for synthesizing the molecules that allow organisms to survive and replicate is encoded in genomic DNA. In the cell, DNA is copied to messenger RNA, and triplet codons (64) in the messenger RNA are decoded - in the process of translation - to synthesize polymers of the natural 20 amino acids. This process (DNA RNA protein) describes the central dogma of molecular biology and is conserved in terrestrial life. We are interested in re-writing the central dogma to create organisms that synthesize proteins containing unnatural amino acids and polymers composed of monomer building blocks beyond the 20 natural amino acids. I will discuss our invention and synthetic evolution of new 'orthogonal' translational components (including ribosomes and aminoacyl-tRNA synthetases) to address the major challenges in re-writing the central dogma of biology. I will discuss the application of the approaches we have developed for incorporating unnatural amino acids into proteins and investigating and synthetically controlling diverse biological processes, with a particular emphasis on understanding the role of post-translational modifications
-
Lecture II: Reprogramming the Genetic Code - part II
TBA
-
Lecture I: Reprogramming the Genetic Code
-
-
Lecture I: Foundational Technologies for Synthetic Biology - from DNA Assembly to Part Characterisation
Synthetic biology is an application-focused field attempting to apply a systematic engineering approach to the design of new biological systems at the molecular level primarily using DNA. Notable progress has been made in the implementation of synthetic genetic circuits in living-systems that mimic functions such as switches, oscillators, timers, pulse generators, band-pass filters and logic gates (1,2). These genetic circuits are anticipated to enable the engineering of biological systems to a complexity previously unattainable. Over the past decade synthetic biology has aided the development of novel biological systems for a range of applications from bacteria that seek and destroy herbicides to the biosynthesis of valuable drugs (3, 4). To accelerate the development of the field many groups are establishing foundational technologies, which can be used across a wide range of applications. Within the Centre for Synthetic Biology and Innovation at Imperial College London (CSynBI; www.imperial.ac.uk/syntheticbiology) we are developing a series of platform technologies to enable the systematic design assembly and testing of synthetic biology designs including integration into an information system. I will describe recent developments in CSynBI on high throughput in vivo part characterization and the automated production of datasheets as well as new approaches in combinatorial DNA assembly. I will also describe our recent in vitro platform for the rapid characterization of DNA regulatory elements using a cell free system (5).
-
Lecture II: Synthetic biology designs for biosensor applications
Biosensors generally can be used to monitor parameters of interest or target analytes and in part they involve a biological component. Early biosensors started when techniques for immobilising enzymes onto solid supports were first developed (1). The relatively recent discovery of bioluminescent (e.g. luciferase) and fluorescent (e.g. green fluorescent protein, GFP) proteins has provided a convenient mechanism for visualising gene expression and protein synthesis in cells and has lead to their use as reporter proteins. By utilizing such constructs, whole cell biosensors have been developed for example to specifically detect certain compounds or act as indicators to monitor cell growth and fitness (2,3). Biological organisms naturally monitor their environment and react accordingly with highly evolved detection and sensing systems, which can now be refactored at the genetic level for specific biosensor applications. One overall aim of synthetic biology is to harness nature's toolbox by fusing molecular biology and engineering disciplines and thus the field of biosensor design and construction has been a major focus for synthetic biologists (4-8). In this lecture I will describe the principles behind biosensor design using synthetic biology approaches providing exemplars to illustrate these principles. I will also present recent data from my groups on developing synthetic biology biosensors for healthcare applications and show how additional societal implications can be integrated into biosensor designs.
-
Lecture I: Foundational Technologies for Synthetic Biology - from DNA Assembly to Part Characterisation
-
-
Lecture I : Genome engineering technologies for rapid editing & evolution organisms
The growing availability of whole genome sequence data sets generated by massively parallel sequencing permits detection of potential associations between genotype and phenotype. These advances motivate the development of high throughput genome editing technologies to systematically elucidate the causative mutations underlying important phenotypes. Similarly, expanding protein and RNA structure databases provide a valuable resource for researchers seeking to engineer macromolecules with new binding specificities. Finally, as complex metabolic networks controlling the flow of biomolecules through cells are elucidated, efforts to alter native metabolism for engineered biosynthesis of desired compounds are rapidly growing in basic research and industrial biotechnology. I will discuss the development of high-throughput and automated methodologies for precise manipulation of genomes. Multiplex automated genome engineering (MAGE) simultaneously targets many locations on the chromosome for modification in a single cell or across a population of cells, thus producing combinatorial genomic diversity. Conjugative assembly genome engineering (CAGE) facilitates the large-scale assembly of many modified genomes. These methods treat the chromosome as both an editable and evolvable template and are capable of fundamentally re-engineering genomes from the nucleotide to the megabase scale. I will present applications of MAGE to generate combinatorial genomic variants from a complex pool of synthetic DNA to diversify target genes in order to optimize biosynthetic pathways. The growing toolbox for genomic engineering will be discussed.
-
Lecture II: Design, construction & function of genomically recoded organisms
The conservation of the genetic code, with minor exceptions, enables exchange of gene function among species, viruses and across ecosystems. Fundamental changes to the genetic code could significantly enhance our understanding of the origins of the canonical code and reveal new subtleties of how genetic information is encoded and exchanged. Modifying the canonical genetic code could also lead to orthogonal biological systems with new properties. I will discuss the construction of a genomically recoded organism (GRO). In the GRO, all known UAG stop codons in Escherichia coli MG1655 were replaced with synonymous UAA codons, which permitted the deletion of release factor 1 and reassignment of UAG translation function. This GRO exhibited improved properties for incorporation of nonstandard amino acids that expand the chemical diversity of proteins in vivo. The GRO also exhibited increased resistance to T7 bacteriophage, demonstrating that new genetic codes could enable increased viral resistance.
-
Lecture I : Genome engineering technologies for rapid editing & evolution organisms
-
Lecture I: Computational protein design - principles, challenges and progress
Designer proteins with new biological functions have tremendous practical importance; they can also enable us to ask questions about the design principles of function and fitness in new ways. Many applications can be imagined: potent protein therapeutics with minimal side effects; new enzymes and biological synthesis pathways for fuel molecules or compounds that are otherwise too expensive to produce; sensor/actuator devices that can report on cell biological processes in real time; robust signaling systems that can detect specific inputs and generate a precise response; protein machines and new biological materials that can be controlled by specific external inputs such as light. The first lecture in this two-part series will describe the current state-of-the-art in computational protein design. I will introduce the main conceptual advances and considerable challenges faced in the field: to sufficiently sample the vast space of possible sequences and structures and to accurately distinguish functional from non-functional designs. Work addressing these challenges uses a combination of ideas from computer science, physical chemistry, engineering and protein biophysics. Highlights from work creating designer proteins - new protein folds, specific binding proteins, and large assemblies - will illustrate where we are.
-
Lecture II: Design of reprogrammed and new functions - from proteins to cells
The second part of the series will focus on current and future opportunities and applications. We'd like to engineer new molecules that operate as predicted in biological contexts, and to utilize prediction and engineering to address fundamental questions on the relationship of molecular characteristics and function. I will describe recent work and new approaches to engineer sensor/actuators that detect and respond to molecules that can currently not be sensed in living cells, and to control the conformational cycle of large protein machines with light.
-
-
Lecture I: Biological Circuit Design by Pareto Optimality
-
Lecture II: Programming Living Molecular Machines for Biofuel Production
-
Lecture I: Biological Circuit Design by Pareto Optimality
-
-
Lecture I: Synthetic Biology of Cell free Systems
The use of parts of cells, specifically enzymes, has a long tradition in therapy, diagnostics and particular chemical synthesis. One of the most attractive feature of enzymes as biological catalysts is that they all have been engineered by nature to operate at comparable environmental conditions. This is a major difference to chemistry and enables the coordinated operation of a cascade of enzymes concomitantly in one vessel, opening ways to efficiently conduct complex chemistry and circumventing thermodynamic challenges. Synthetic biology allows constructing such systems with an unprecedented degree of scope and ease. We will illustrate the potential of the approach by discussing the generation of large enzyme systems for the production of fine chemicals. Furthermore, we will discuss an engineering approach to optimizing such systems based on advanced on-line MS-analytics and design of experiments.
-
Lecture II: Exploiting Engineered Cell-Cell Communications in Large Scale Biotechnology
Synthetic biology brings with it a drastic increase in the scope of experimental operations, which it typically accommodated by a tendency to miniaturize and parallelize experimentation. While this is relatively straight forward for in vitro approaches such as DNA assembly, suitable approaches are less clear when interaction of living cells is required. We will discuss recent efforts of our laboratory to develop nanoliter-sized cultivation systems and their resulting applications to high-throughput screening strategies in the fields of fluorescent protein engineering, vitamin production, and engineering of novel antibiotics.
-
Lecture I: Synthetic Biology of Cell free Systems
-
-
Lecture I: Analog versus Digital Computation in Biology
The fundamental laws of noise in gene and protein expression set limits on the energy, time, space, molecular count, and part-count resources needed to compute at a given level of precision in the cell. Based on these laws, we conclude that analog computation is significantly more efficient in its use of resources than deterministic digital computation in the cell. Hence, synthetic and natural circuits in cells must use analog, collective analog, probabilistic, and hybrid analog-digital computational approaches to function; otherwise, even relatively simple computations in cells like addition will exceed energy and molecular-count budgets. We present schematics for efficiently representing analog DNA-protein computation in cells. A deep connection between analog circuits and cell biology enables us to also engineer synthetic analog computation in cells efficiently.
-
Lecture II: Analog Synthetic and Systems Biology
The deep connection between analog circuits and cell biology arises because there are astounding similarities between the equations that describe noisy electronic flow in sub-threshold transistors and the equations that describe noisy molecular flow in chemical reactions, both of which obey the laws of exponential thermodynamics. Based on these similarities, we have engineered logarithmic analog computation in living cells with less than three transcription factors, almost two orders of magnitude more efficient than prior digital approaches. In addition, highly computationally intensive noisy DNA-protein and protein-protein networks can be rapidly simulated in mixed-signal supercomputing chips that naturally capture their noisiness, dynamics, and loading interactions at lightning-fast speeds. Such an approach may enable large-scale design and analysis in synthetic and systems biology that is faithful to how messy analog biology works, quite different from clean, well-defined digital design.
-
Lecture I: Analog versus Digital Computation in Biology
-
-
Lecture I: Minimal Genomes: High-Throughput Sequencing, Statistical Methods and Physics Models to Unveil Minimal Yeast Chromosomes Compatible with Life
-
Lecture II: Computational Tools for Genome editing, Combinatorial Assembly and Workflow Tracking
-
Lecture I: Minimal Genomes: High-Throughput Sequencing, Statistical Methods and Physics Models to Unveil Minimal Yeast Chromosomes Compatible with Life
-
-
Lecture I: Synthetic Biology: From Parts to Modules to Systems
-
Lecture II: Mammalian Synthetic Biology: Scientific and Therapeutic Applications
-
Lecture I: Synthetic Biology: From Parts to Modules to Systems
-
- Lecture I:
Synthetic biology aims at engineering systems from characterized genetic parts to perform complex coordinated tasks for pharmaceutical and industrial applications. Mammalian synthetic biology slowly enters the phase where proof-of-concept designs move closer to therapy. Engineered networks are designed to sense clinically relevant molecules, integrate signals from the various sensors and finally produce a clinically relevant output. A key feature is the ability of such synthetic circuits to "sense" intracellular states or exogenous cues. We will discuss designs of sensory circuits for metabolites of human body, proteins, mRNA and microRNA.
- Lecture II:
Another critical aspect on the way of mammalian synthetic biology towards therapy is safety of the engineered systems. We will discuss different approaches undertaken to ensure the minimal negative footprint of the engineered systems on the host organism including cell-based therapies, and circuits encoded exclusively on RNA. Additionally we will discuss development of platforms for easy prototyping of genetic circuits that can be used to test their long term behavior and reliability.
Workshop on "Biosensors and Synthetic Circuits in Mammalian Cells"
- Lecture I:
SSBSS is organized by the TaoSciences Research Center
SSBSS 2014 Directors
- Jef Boeke, Johns Hopkins University, USA
- Giuseppe Nicosia, University of Catania, Italy
- Mario Pavone, University of Catania, Italy
- Giovanni Stracquadanio, Johns Hopkins University, USA
School Director
Scientific Committee
- Giuseppe Nicosia, University of Catania, Italy
- Joel Bader, Johns Hopkins University, USA
- Jole Costanza, University of Catania, Italy
- Barbara Di Camillo, University of Padova, Italy
- Markus Herrgard, Novo Nordisk Foundation Center for Biosustainability, Technical University of Denmark, Denmark
- Heiko Muller, Italian Institute of Technology, Italy
- Giuseppe Narzisi, Cold Spring Harbor Laboratory, USA
- Wieslaw Nowak, Nicholas Copernicus University, Poland
- Gianna Maria Toffolo, University of Padova, Italy
- Renato Umeton, MIT, USA
- Luca Zammataro, Italian Institute of Technology, Italy
Sponsors
Platinum Sponsors:

Gold Sponsors:
Leonardo Design Systems
Silver Sponsors:
Bronze Sponsors:
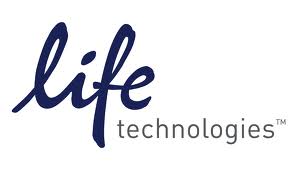
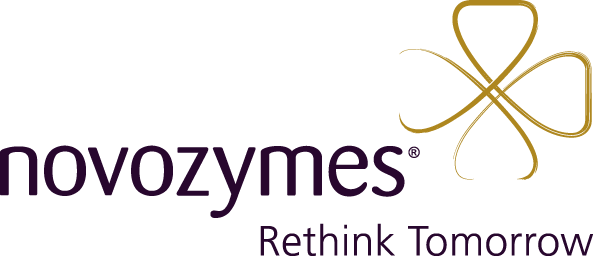